Enzyme activity and active site analysis are critical for understanding how enzymes behave as biological catalysts. Their activity is affected by temperature, pH, substrate concentration, and the presence of inhibitors or activators. Each enzyme requires an optimum environment for optimal operation, and departures from this can impair its activity. The active site, which is responsible for substrate binding and catalysis, is critical to enzyme specificity and efficiency. The active site is investigated using techniques like as X-ray crystallography and site-directed mutagenesis, which provide insights into enzyme mechanisms and facilitate advances in domains such as medicine and biotechnology.
Table of Contents
Enzyme Activity
Enzyme activity is the rate at which an enzyme catalyzes a chemical process. Enzymes play an important role in biochemistry because they facilitate a variety of metabolic reactions that are required for life. Several factors affect enzyme activity, such as substrate concentration, temperature, pH, and the presence of inhibitors or activators. Enzymes are biological catalysts that speed up chemical reactions in living organisms. Their activity can be influenced by several factors:
Temperature Effects
Temperature is a critical factor that influences enzyme activity. The relationship between temperature and enzyme function can be summarized as follows:
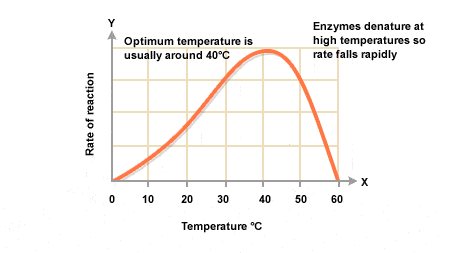
Optimal Temperature: Each enzyme has a specific temperature at which it performs best, known as the optimal temperature. For many human enzymes, this is around 37°C (98.6°F). At this temperature, the enzyme’s structure is stable, and the reaction rate is maximized.
Increasing Temperature: As the temperature rises towards the optimal level, enzyme activity typically increases. This is due to the enhanced kinetic energy of molecules, which leads to more frequent collisions between enzymes and substrates. Consequently, the rate of enzyme-catalyzed reactions increases.
Denaturation at High Temperatures: If the temperature exceeds the optimal range (usually above 40-50°C for many enzymes), the enzyme may undergo denaturation. This process involves the alteration of the enzyme’s three-dimensional structure, particularly the active site, which prevents it from binding to its substrate effectively. Denaturation can result in a complete loss of enzyme function.
Low Temperatures: At low temperatures (typically below the optimal range), enzyme activity decreases. The reduced kinetic energy results in fewer collisions between enzymes and substrates, leading to slower reaction rates. However, enzymes do not denature at low temperatures; they simply become less active.
pH Effects
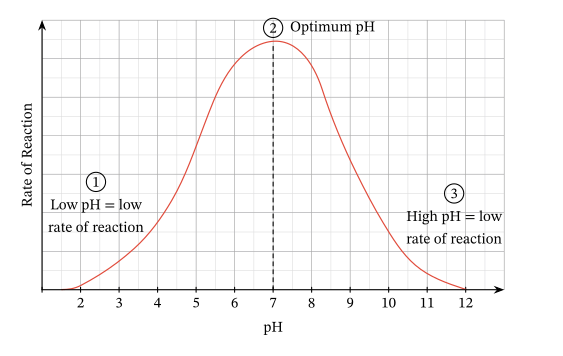
Ionization of amino acids: The ionization state of amino acid side chains in the active site has a significant effect on enzyme activity. This is due to the charge and hydrogen bonding properties of these side chains, which are essential for substrate binding and catalysis.
Optimal pH: Each enzyme has a specific pH range where it performs best. Pepsin, found in the stomach, performs best at a pH of around 2 in acidic conditions, whereas trypsin, found in the small intestine, performs best at a pH of about 8 in slightly alkaline conditions. This optimal pH is critical for the enzyme’s structural and functional integrity.
Extreme pH
Denaturation: Extreme pH values, whether too acidic or too alkaline, can disrupt the enzyme’s 3D structure.
Loss of Function: Disruption of the enzyme’s structure, including the active site, leads to loss of function.
Active Site Studies
The active site is the specific region of an enzyme where substrate binding and catalysis take place.
Identification of Binding and Catalytic Sites
X-ray Crystallography: This technique enables researchers to determine the enzyme’s 3D structure, revealing the precise location of the active site and the amino acid residues involved in substrate binding and catalysis.
Site-Directed Mutagenesis: Scientists can explore the relevance of certain amino acids in enzyme action by modifying them in the active site. This approach aids in identifying the residues responsible for substrate binding and catalysis.
Trapping the Enzyme-Substrate Complex
Cryotrapping: Rapid freezing maintains the structure of the enzyme-substrate complex, allowing researchers to analyze its conformation and interactions at atomic resolution using techniques such as cryo-electron microscopy.
Cross-Linking: This involves chemically binding the enzyme and substrate together, stabilizing the complex and allowing for comprehensive study.
Use of Substrate Analogues
Substrate analogues are molecules that resemble natural substrates but have slight differences. Scientists use them to study how enzymes recognize and bind to their substrates.
Competitive Inhibitors: These analogues mimic the original substrate and compete for the enzyme’s active site. By blocking this location, they prevent the real substrate from binding, allowing researchers to better understand enzyme specificity.
Transition State Analogues: These represent a transitional state during the response. They attach to the enzyme more closely than the actual substrate, effectively inhibiting its function. This helps scientists understand how enzymes work.
Enzyme Modification by Chemical Procedures
Researchers can modify specific amino acids in enzymes to better understand their roles in enzyme activity. Here are some common methods:
Chemical Modification: This involves modifying the side chains of certain amino acids with chemical reagents. This allows scientists to discover which amino acids are required for binding and catalysis.
Proteolytic Cleavage: This approach disrupts peptide links to reveal the enzyme’s critical areas. Researchers can establish which parts are critical to the system’s function by studying the generated fragments.
Site-Directed Mutagenesis: This approach causes precise mutations in the enzyme’s DNA. Scientists can learn about the significance of specific amino acids by investigating how these mutations affect enzyme performance.
Enzyme Modification by Treatment with Proteases
Proteases: These are enzymes that degrade proteins. Researchers can use proteases to remove certain sections of an enzyme to examine how this affects its activity, so identifying critical regions.
Site-Directed Mutagenesis
Mutagenesis: This is a method used to create specific changes in the DNA sequence of an enzyme, leading to changes in the amino acid sequence. By studying these mutations, scientists can determine the roles of specific amino acids in enzyme function.
Conclusion
Enzymes are important biological catalysts whose activity is strongly regulated by temperature and pH, with optimal conditions required to maintain their structural integrity and function. Active site studies, which involve techniques such as X-ray crystallography and site-directed mutagenesis, improve our understanding of substrate binding and catalytic mechanisms, whereas cryotrapping and the use of substrate analogues provide information about enzyme specificity and interactions. Furthermore, enzyme modification via chemical methods and protease treatment enables researchers to discover essential amino acids required for enzymatic activity. Collectively, these studies not only add to our understanding of enzymatic activities, but they also have practical applications in medicine, biotechnology, and drug discovery, ultimately enhancing our grasp of molecular biology.
Frequently Asked Questions (FAQ)
What are enzymes, and why are they important?
Enzymes are biological catalysts that accelerate chemical reactions in living systems. They serve an important part in the many metabolic processes required for life, allowing reactions to occur more quickly.
How do temperature and pH affect enzyme activity?
Enzymes perform best at appropriate temperatures and pH levels. Deviations from these parameters might result in diminished activity or denaturation, which alters the enzyme’s structure and prevents it from operating properly.
What is the active site of an enzyme?
The active site is a specific area of the enzyme where substrate molecules attach and undergo a chemical reaction. It is important for enzyme selectivity and catalytic efficiency.
How can scientists study the active site of an enzyme?
Researchers utilize techniques like X-ray crystallography to establish the 3D structure of enzymes and pinpoint their active sites. Site-directed mutagenesis allows scientists to change specific amino acids to better understand their functions in substrate binding and catalysis.