The human genome, a complex tapestry of DNA containing the blueprints for life, is not as static as it might seem. Embedded within its intricate structure are mobile elements, known as transposable elements (TEs) or “jumping genes,” capable of moving around the genome. These enigmatic sequences, though once considered “junk DNA,” are now recognized as dynamic players in shaping the evolution and function of our genetic landscape.
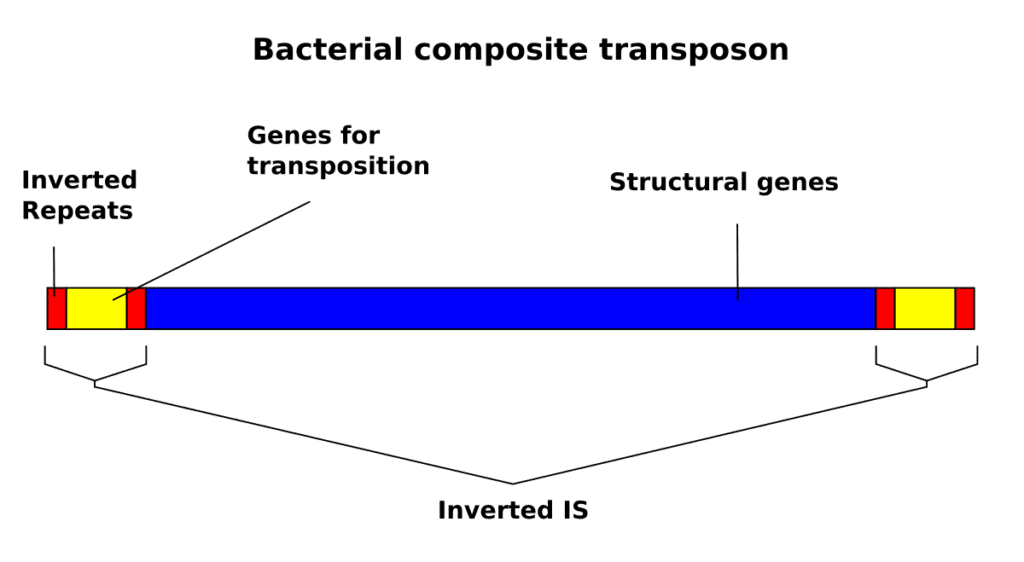
Table of Contents
The Discovery of Jumping Genes
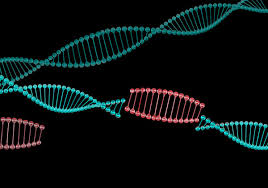
The discovery of Transposable elements as a revolutionary moment in biology, challenging the prevailing dogma of a fixed and immutable genome. Barbara McClintock’s pioneering work in the 1940s and 50s with maize (corn) revealed the existence of these mobile genetic elements. She observed unstable patterns of pigmentation in corn kernels, a phenomenon caused by the movement of TEs, which she termed “controlling elements.” Her groundbreaking research, though initially met with skepticism, earned her the Nobel Prize in Physiology or Medicine in 1983, solidifying the importance of TEs in genetic processes.
Types of Transposable Elements
TEs are broadly classified into two major classes:
Class I Transposable elements (Retrotransposons)
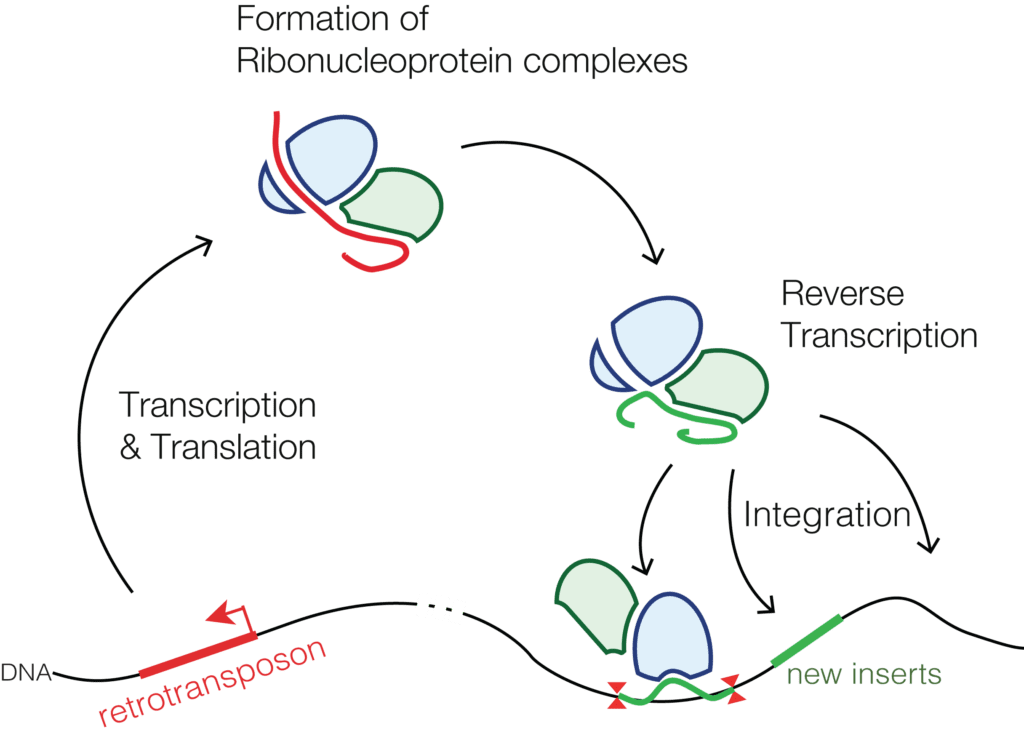
These elements move via an RNA intermediate. They “copy and paste” themselves, transcribing their DNA into RNA, which is then reverse transcribed back into DNA by a specialized enzyme called reverse transcriptase. This newly synthesized DNA copy integrates into a new location in the genome. Retrotransposons are further divided into:
Long Terminal Repeat (LTR) Retrotransposons: These elements are characterized by long, repeated sequences at their ends, known as LTRs. They often contain genes encoding for reverse transcriptase and other proteins required for their transposition. Examples include Ty elements in yeast and copia elements in Drosophila.
Non-LTR Retrotransposons: These elements lack LTRs and utilize a different mechanism for transposition. The most prominent example in humans is the LINE (Long Interspersed Nuclear Element) family, which makes up a significant portion of our genome.
Class II Transposable elements (DNA Transposons)
These elements move directly as DNA, using a “cut and paste” mechanism. They excise themselves from their original location and insert into a new site in the genome. This process involves the action of a transposase enzyme, which facilitates the movement. Examples include the Ac/Ds elements in maize and the P elements in Drosophila.
Transposable elements: A Double-Edged Sword
The presence of Transposable elements in the genome poses both a challenge and an opportunity for cellular function:
Challenges
Genetic Instability: TE movement can disrupt gene expression, leading to mutations and genomic rearrangements, potentially causing diseases.
Disease Association: Some TEs have been implicated in various human diseases, such as cancer, neurodevelopmental disorders, and immune disorders.
Antibiotic Resistance: TEs can transfer antibiotic resistance genes between bacteria, contributing to the spread of drug-resistant infections.
Opportunities
Genetic Diversity: TEs contribute to the generation of genetic diversity within populations, providing raw material for natural selection and evolution.
Novel Gene Regulation: TEs can create new regulatory elements, altering gene expression patterns and contributing to the complexity of cellular processes.
Genome Evolution: TEs have played a significant role in shaping the structure and function of genomes throughout evolutionary history.
TEs and the Human Genome
The human genome is estimated to be approximately 45% composed of transposable elements, primarily retrotransposons. While these elements have been largely silenced over time, they still retain the potential to transpose, albeit at low frequencies. However, even silent TEs can influence gene expression by providing binding sites for transcription factors or by affecting chromatin structure.
Controlling TE Activity
Cells have evolved sophisticated mechanisms to suppress TE activity and prevent their uncontrolled transposition. These include:
Epigenetic Silencing: TEs are often silenced by methylation, a chemical modification of DNA that inhibits gene expression.
Small RNAs: Small RNA molecules can target TE transcripts, triggering their degradation and preventing their transposition.
Chromatin Remodeling: Chromatin structure, the packaging of DNA around histone proteins, can be modified to restrict TE activity.
TEs in Research and Biotechnology
Despite their potential disruptive nature, TEs are becoming increasingly valuable tools in research and biotechnology:
Genetic Engineering: TEs can be harnessed to precisely insert genes into genomes, allowing for gene therapy and genetic modifications.
Evolutionary Studies: Studying the distribution and evolution of TEs can provide insights into the evolutionary history of species and their adaptations.
Disease Modeling: TEs can be used to create animal models of human diseases, facilitating the study of disease mechanisms and the development of new therapies.
Conclusion
Transposable elements are not simply “junk DNA,” but dynamic components of our genome, shaping its evolution and function. Their movement, although potentially disruptive, has contributed to the diversity and complexity of life. As research continues to unravel the mysteries of TEs, we are gaining a deeper understanding of their roles in health, disease, and the evolution of life itself.
Frequently Asked Questions(FAQ)
What do you mean by Junk DNA?
The phrase “junk DNA” refers to non-coding DNA sequences, which do not transcribe into proteins, and is a remnant of the past. Scientists thought these sequences were useless evolutionary leftovers for many years. But as research shows how important these sequences are to cellular function, this view is quickly shifting.
Define RNA?
A versatile molecule that is necessary for life is RNA. It ensures that our cells are working properly by serving as a link between DNA and protein production.
Related Articles